Oncological diseases
Personalised diagnostics for optimal therapy prediction
In the field of expertise biospectroscopy, novel vibration spectroscopic methods are being developed, to diagnose cancer diseases more precisely and earlier. To this end, we examine tissue thin sections and cell sediments using using spectroscopic imaging techniques. We call this approach label-free digital pathology (Großerüschkamp, Gerwert GIT Laboratory Journal 2018).
If we look at colorectal carcinoma, for example, immunotherapy can already be used today in advanced stages. This involves marking the cancer cells specifically for the body’s own immune system so that our own body can break them down. However, this form of therapy is not effective for every tumour patient. Today it is still possible that one patient receives this therapy but does not benefit from it, while another patient who would benefit from it does not receive it. The reason for this is that some of the diagnostic tools available are not yet suitable for fully recording all the individual characteristics of the patients. Only precise, personalised diagnostics can predict the best therapy for each patient. Every person is unique.
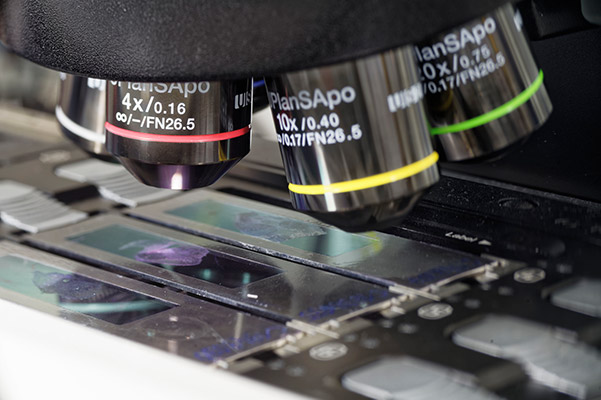
Figure 1: Classical histology – Thin tissue sections are stained and examined by an experienced pathologist using a light microscope.
Novel diagnostic methods through spectroscopy
In the competence area Biospectroscopy we identify and validate characteristic vibration spectroscopic biomarkers to automate the diagnosis and classification of oncological diseases. Due to its high sensitivity and the cumulative detection of all substances contained, a vibration spectrum represents a complete biochemical image of the tissue sample – like a fingerprint. The spectra are recorded in just a few seconds without staining the sample or chemically marking components (Kuepper et al Scientific Reports 2018).
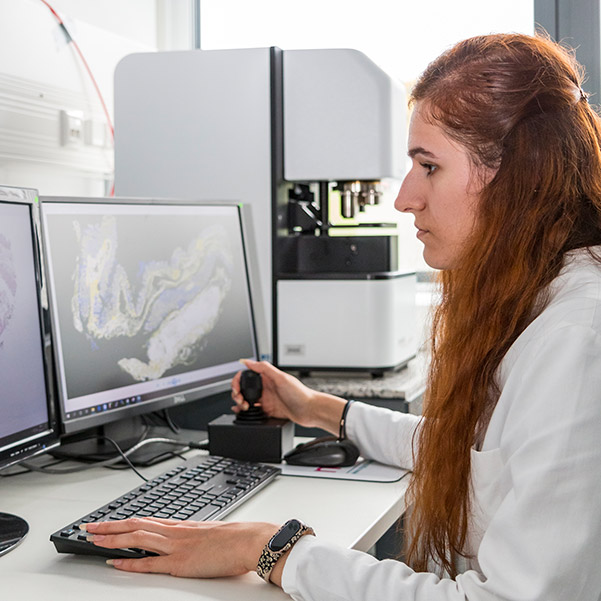
Figure 2: Researcher at a modern IR imaging system. The screen shows the false-colour image of the tissue in IR imaging.
Spectroscopy in cancer research
For this purpose we analyse tissue and body fluids. Tissue samples are analysed using IR (InfraRed) and Raman spectroscopic methods. Both spatially resolved microspectroscopic approaches and point measurements with fibre probes are used. The spatially resolved measured spectra are grouped according to spectral similarity and displayed as false-colour images for microspectroscopy. The higher spatial resolution of the Raman technique even allows three-dimensional imaging of intracellular components on living cells (Hammoud et al Scientific Reports 2018). After pathological annotation, classifiers are trained to identify tissue and pathological changes automatically in the future, exclusively on the basis of spectral signatures, and to support doctors in their diagnostics.
This automated and sample-conserving method allows consistently precise cancer diagnostics even on the smallest biopsies without destroying the sample for subsequent examinations such as proteome analyses (Großerüschkamp et al Scientific Reports 2017). Research in the field of expertise biospectroscopy focuses on colon, bladder and thoracic tumours (e.g. lung cancer). Here, we have succeeded in correlating even therapy-relevant molecular changes in the tumours with spectral fingerprints. This allows a fast, therapydecisive diagnostic aid that can diagnose cancer with consistent accuracy, regardless of a person’s experience.
The effect of drugs
In addition, our approach enables research into the mechanisms of action of drugs. For example, cancer drugs can be tracked in individual cells, allowing important conclusions to be drawn about their mode of action (Yosef et al Analyst 2018).In addition to our diagnostic approach, this helps pharmaceutical researchers to develop more precise active ingredients. In combination with precise diagnostics, this allows for a gentle and successful treatment of patients (Aljakouch et al Analytical Chemistry 2019).
Types of oncological diseases
Colon cancer (e.g. colorectal carcinoma)
Colorectal cancer (CRC) is the third most common type of cancer and one of the leading causes of cancer-related death worldwide. In recent years, many improvements in treatment have been based on a deeper understanding of molecular heterogeneity at several levels, including genomics, epigenomics and transcriptomics. For some patients, novel drugs such as pembrolizumab or nivolumab/pilimumab can already be used today. This method is also known as immunotherapy, as it allows advanced (stage IV) CRC to make tumour cells vulnerable to the body’s own immune system by use of antibodies. Although this gentle therapy is very expensive, it is extremely effective; at least in some patients. The correct diagnose of these patients, who respond well to immunotherapy, is one of the goals of our colon cancer project.
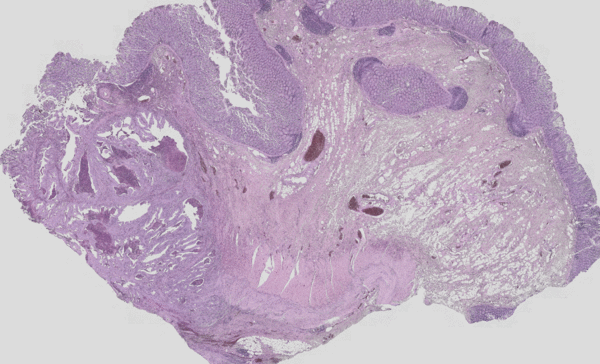
Figure 3: Classical H&E staining (red-violet-blue staining) of a tumour superimposed on the IR imaging false colour image. On the left, the tumour lesion is clearly visible as an unstructured area in the H&E image. In IR imaging, this area has been automatically marked in red without the user’s prior knowledge. The structure of a rather healthy intestine can be seen on the right side. The IR imaging allows the analysis of all tissue types.
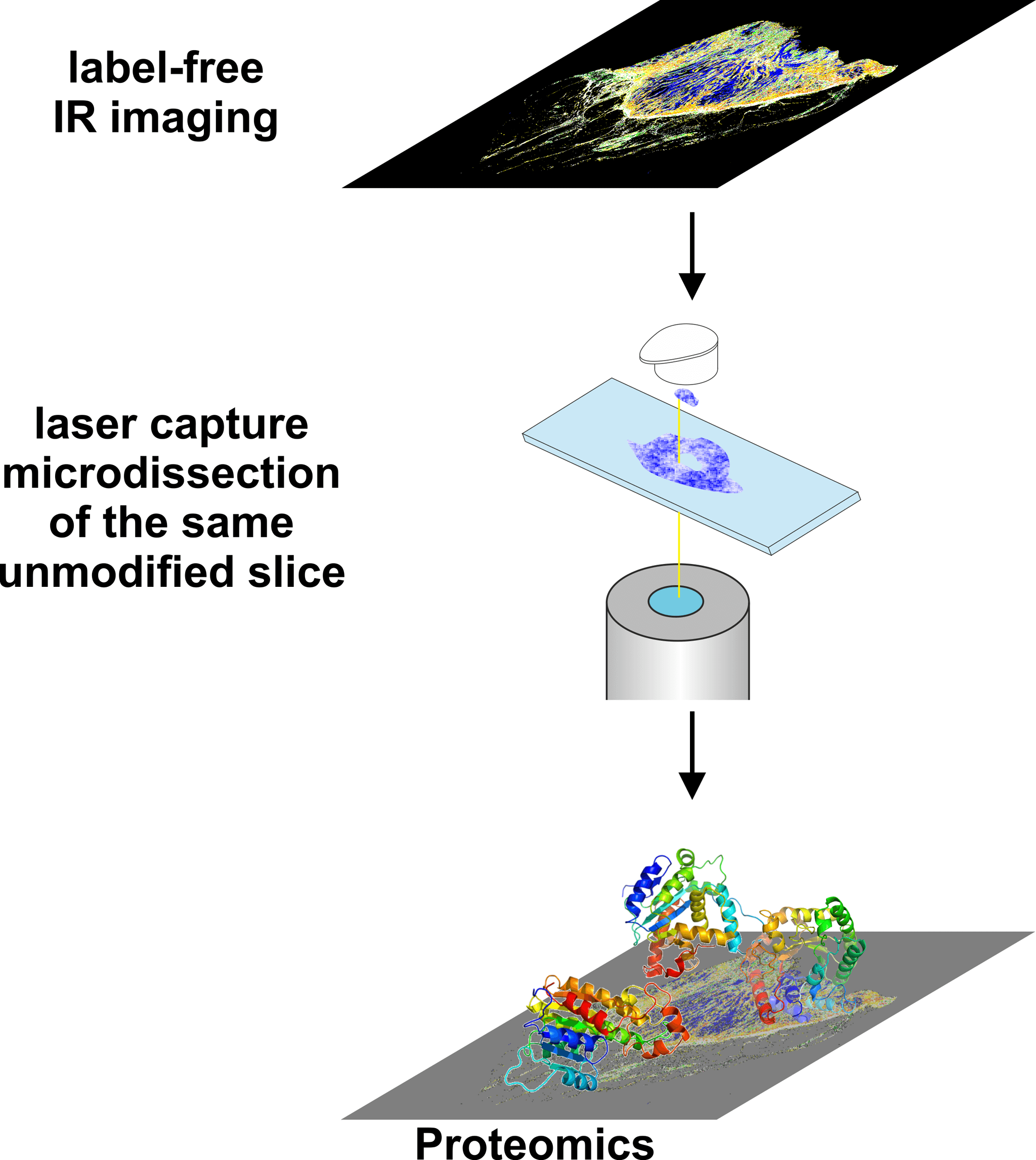
Figure 4: Label-free recognition by IR imaging allows the precise selection of tissue areas for subsequent examinations, such as proteomics.
In cooperation with the clinics of the Ruhr-University Bochum and the other competence areas, we are investigating colorectal carcinoma (Kuepper et al Faraday Discussions 2016, Kallenbach-Thieltges et al Journal of Biophotonics 2013). For this purpose we have created a spectral database (Kuepper et al Scientific Reports 2018). With FTIR and Raman imaging we were able to establish a characterisation of thin tissue sections of the intestine with high accuracy (>96 %). Finest details, such as the muscularis mucosae, which is important for the classification of carcinoma, can be resolved (Kuepper et al Scientific Reports 2018). The next steps in this project will focus on the validation of the methodology using the Colopredict Plus 2.0 registry study and the mapping of molecular changes that are crucial for therapy. The primary goal is to translate this innovative methodology into clinical use.
Bladder cancer / prostate carcinoma
Bladder cancer is also examined in cooperation with the clinics of the Ruhr University Bochum. In the current project, IR imaging was used to establish the automated characterisation of bladder tumours. A central, further-reaching issue in the diagnosis of bladder cancer is the differential question of the degree of tumour. This is the decisive factor for the removal of the bladder. The diagnosis of bladder cancer is sometimes difficult, since an inflammation of the bladder is very difficult to distinguish from a high grade carcinoma (carcinoma in situ – CIS). By using IR-imaging we were able to establish an automated characterisation of the tissue, which can answer the differential question of the tumour grade. This very precise and robust characterisation is currently being validated. This will help to avoid perspectively unnecessary resections of the bladder and the associated suffering (Witzke et al The American Journal of Pathology 2019).
Through combination with omics techniques, a deeper understanding of the biochemistry of bladder tumours will be gained. A new biomarker (AHNAK2) for the diagnosis of CIS in ProDi has already been identified (Witzke et al The American Journal of Pathology 2019).
In addition, we are developing a non-invasive method for the early detection and diagnosis of bladder cancer in the competence area Biospectroscopy. Raman techniques are used to characterise the cell components of urine (El-Mashtoly, Gerwert GIT Imaging & Microscopy 2019). This approach is expected to reduce the number of cystoscopies and thus improve the readiness for prevention.
In the coming years this approach will be extended to prostate carcinomas. In this case, determining the degree of tumour is one of the decisive factors in therapy and should be simplified and improved by our innovative approach.
Through combination with omics techniques, a deeper understanding of the biochemistry of bladder tumours will be gained. A new biomarker (AHNAK2) for the diagnosis of CIS in ProDi has already been identified (Witzke et al The American Journal of Pathology 2019).
In addition, we are developing a non-invasive method for the early detection and diagnosis of bladder cancer in the competence area Biospectroscopy. Raman techniques are used to characterise the cell components of urine (El-Mashtoly, Gerwert GIT Imaging & Microscopy 2019). This approach is expected to reduce the number of cystoscopies and thus improve the readiness for prevention.
In the coming years this approach will be extended to prostate carcinomas. In this case, determining the degree of tumour is one of the decisive factors in therapy and should be simplified and improved by our innovative approach.
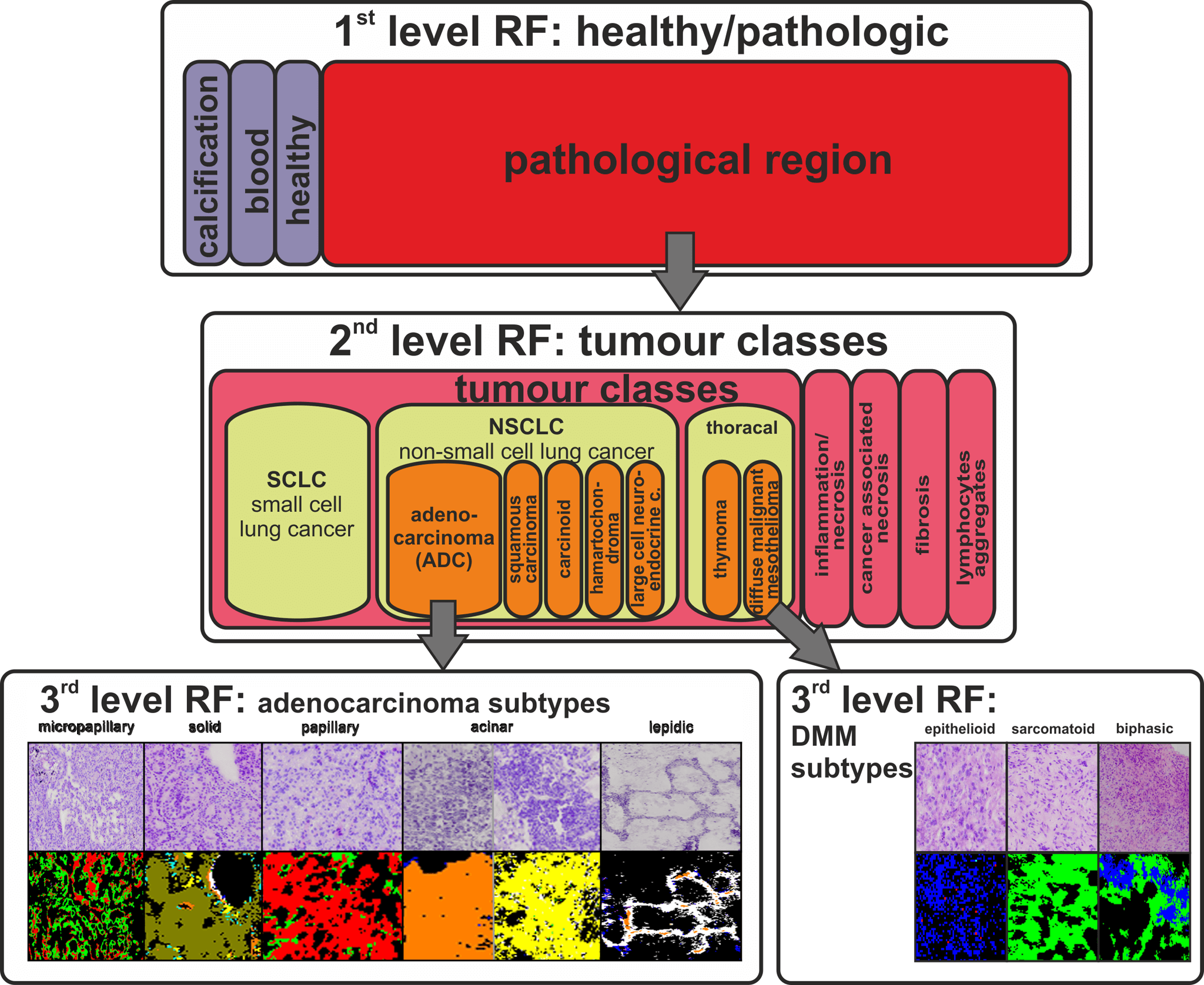
Thoracic tumours
Lung cancer is one of the cancers with the worst prognosis for patients with the disease. This is partly due to the high complexity of the disease and also to the often very late diagnosis of a lung tumour. The aim of our projects is to find diagnostic markers to characterise lung cancer. With FTIR imaging we were able to establish an automated characterisation of lung cancer and immigrating tumours (e.g. mesotheliomas of the pleura) with an accuracy of > 94 % (Großerüschkamp et al Analyst 2015). In order to increase the prognostic value of this detection, it was extended to include the detection of tumour subtypes. Thus, we were the first group worldwide to be able to establish an automated detection of the subtypes of adenocarcinomas of the lung on thin tissue sections. In descending order of mortality, these are micropapillary, solid, papillary, acinar and lepidic carcinoma. Until now, the assignment of these subtypes was only possible by means of complicated immunohistochemical staining methods with a high susceptibility to errors.
Due to the high heterogeneity of lung tumours, the acquisition of biochemical information or even biomarker candidates is very difficult, especially in this entity. Our approach, combining FTIR imaging with laser microdissection to obtain samples for omics techniques, will enable us to obtain samples for tissue analysis with unprecedented accuracy. First promising experiments show that this approach allows a very precise molecular characterisation of the tissue and may provide new biomarker candidates (Großerüschkamp et al Scientific Reports 2017).
Companion diagnostics of active substances
Evaluation of targeted cancer therapies by Raman microscopy
The evaluation of the effectiveness of drug candidates in vitro is essential in the early stages of drug development. The in vitro tests currently used to monitor drug efficacy are often performed with fluorescence-labelled drug molecules. Despite the high molecular specificity offered by fluorescent molecules, they are often much larger than the drug molecules themselves and can significantly influence the pharmaceutical activity of the drug. Alternatively, we have developed a label-free method using human cell lines treated with drug candidates. Both conventional Raman microscopy and stimulated Raman scattering (SRS) can be used as in vitro screening methods.
To determine the efficacy of drugs at the subcellular level, we first established an automatic identification of cellular organelles including the nucleus, nucleolus, lipid droplets, endoplasmic reticulum, Golgi apparatus and mitochondria. Raman microscopy is also used to identify the cellular response to targeted cancer therapies, such as EGFR inhibitors. For example, the influence of certain driver protein mutations, such as the oncogenic K-Ras protein or EGFR, can be investigated. Furthermore, the spatial distribution and metabolism of tyrosine kinase inhibitors such as erlotinib or neratinib in cancer cells can be demonstrated. Thus, Raman microscopy offers great potential for pharmacodynamics and pharmacokinetics studies in cells.
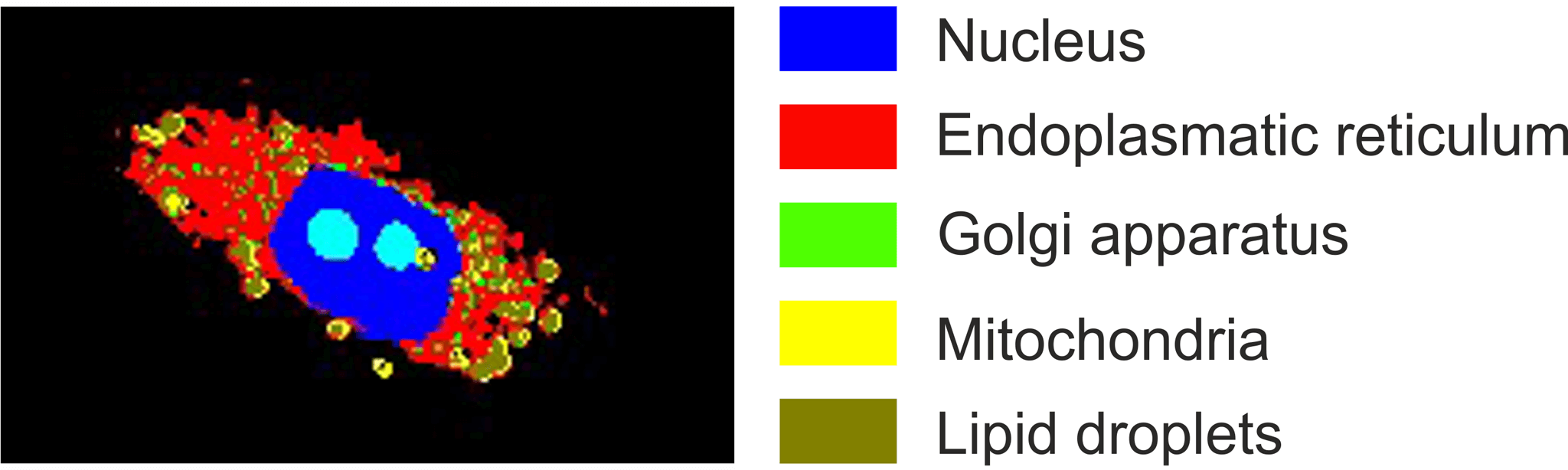
Figure 6: Label-free identification of cell organelles
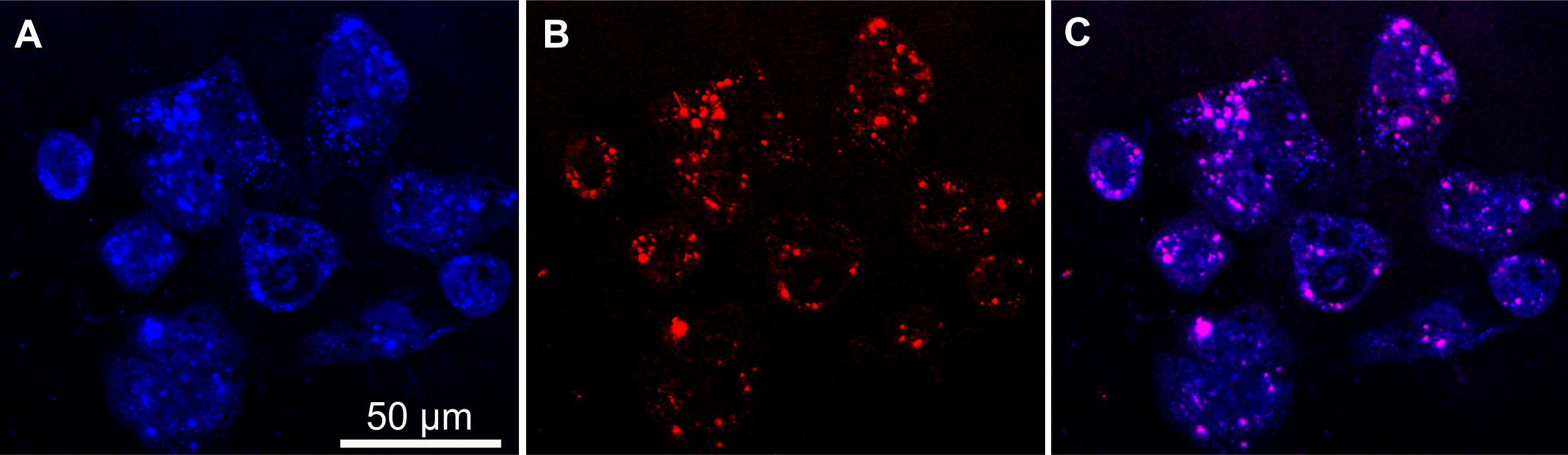
Figure 7: SRS intensities of (A) the C-H stretch vibration, indicative of lipid and proteins of cells and (B) C≡N stretch vibration, marker of neratinib and (C) superposition of (A) and (B).
Press
Presse releases
Press review
External Funding
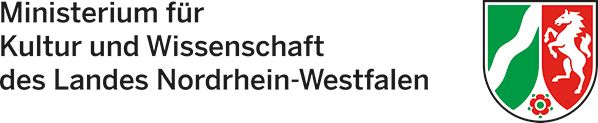
111.08.03.05-133974
Validation and standardization of the protein diagnostics workflow
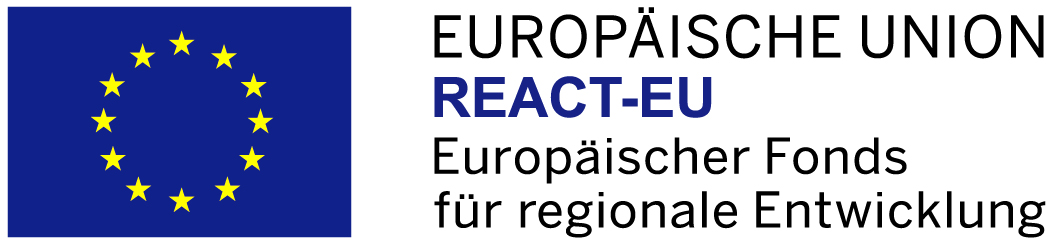

EFRE funding code: EFRE-0802016
Recovery Assistance for Cohesion and the Territories of Europe (REACT-EU) – Call for projects “Digitisation in clinical medical and health research”
FP-0259 CDE
Verification of novel molecular markers for early diagnosis of lung tumors for the benefit of follow-up screening.
FP-0241 BC
Marker-assisted follow-up of patients with non-muscle-invasive low/intermediate-risk urinary bladder tumors (UroFollow)
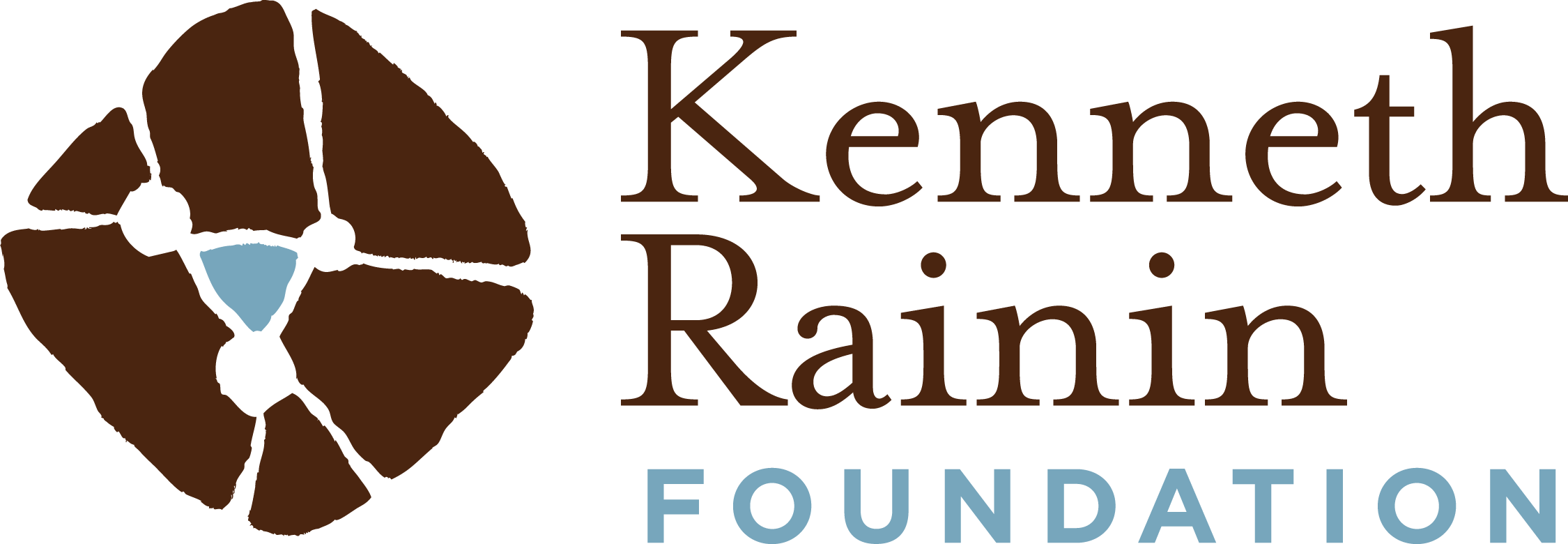
Label-free digital pathology and spatially resolved transcriptomics for the identification of novel biomarkers and therapeutic targets in inflammatory bowel diseases
131/1.08-031
PURE – Protein Research Unit Ruhr within Europe
Validation of marker-free imaging methods and newly identified biomarkers using the PURE consortium
FP-339
Development of protein analytical methods for the identification of candidate markers to support the (early) diagnosis of asbestos-associated lung and pleural tumors.
Key Publications
Großerüschkamp, Gerwert GIT Laboratory Journal 2018
Colon Cancer
Kuepper et al Scientific Reports 2018
Kuepper et al Faraday Discussions 2016
Kallenbach-Thieltges et al Journal of Biophotonics 2013
Lung Cancer
Großerüschkamp et al Scientific Reports 2017
Großerüschkamp et al Analyst 2015
Bladder cancer
Witzke et al The American Journal of Pathology 2019
Companion Diagnostics
Hammoud et al Scientific Reports 2018
Aljakouch et al Angewandte Chemie 2018
El-Mashtoly et al Analytical Chemistry 2015