Analysis of body fluids
Minimally invasive diagnostics
Immuno-Infrared Sensor
In addition to tissue-based imaging approaches for the identification of new biomarker candidates and for the precise (early) diagnosis of oncological diseases, the competence area biospectroscopy also focuses in particular on human body fluids such as blood plasma and cerebrospinal fluid (CSF) for the (early) diagnosis of neurodegenerative diseases. Diagnostics on body fluids has the great advantage that blood in particular can be obtained in a minimally invasive and cost-effective manner as part of routine diagnostics both in clinics and in general practitioners’ offices.
Biomarker detection for neurodegenerative diseases
Today’s widely used quantitative methods for the detection of specific biomarker candidates in body fluids, such as enzyme-linked immune-sorbent assays (ELISA) or mass spectrometric techniques, have not yet become widely accepted for blood tests for neurodegenerative diseases. These techniques either detect the total amount of a protein regardless of its folding or selectively detect only a single secondary structure. Since neurodegenerative diseases are usually associated with protein misfolding, measurement of the total distribution of secondary structures of disease-associated proteins, such as amyloid-β (Aβ) peptide in Alzheimer’s disease (AD), is desirable.
Infrared spectroscopy provides information about misfolding
We have developed a method that detects all Aβ-peptides and simultaneously analyses their secondary structure distribution using infrared (IR) spectroscopic measurement techniques. An increased proportion of β-sheet-rich structures in the overall distribution of all Aβ-peptides is associated with Alzheimer’s disease. Using IR spectroscopy, information about the secondary structure distribution of proteins is obtained much faster and easier than with X-ray crystallography or nuclear magnetic resonance (NMR) spectroscopy. The most characteristic and largest absorption band of proteins is the amide I band, which is caused by absorption of the peptide backbone and lies between 1700 und 1600 cm-1. The exact position of the amide I band depends on the respective secondary structure (Nabers et al Journal of Biophotonics 2016). The C=O stretching vibration of the peptide bond accounts for the largest proportion of the absorption of the amide I band. The differences in the hydrogen bonds between C=O and NH groups and the couplings of the C=O vibrations lead to different vibrational energies and thus to different absorption frequencies of the amide I bands. The secondary structure β-sheet, in which the Aß fibrils associated with AD are mainly present, elicits an amide I band with a maximum at 1630 cm-1 hervor. In contrast, α-helical and disordered secondary structures of proteins absorb in the range of 1655 – 1645 cm-1. Since healthy people have a high proportion of disordered / α-helical Aβ-peptides, their samples can be distinguished from those of patients with Alzheimer’s disease by the shape of the amide-I band. For this to work, however, the entire distribution of the secondary structures of all Aβ-peptides must be measured.
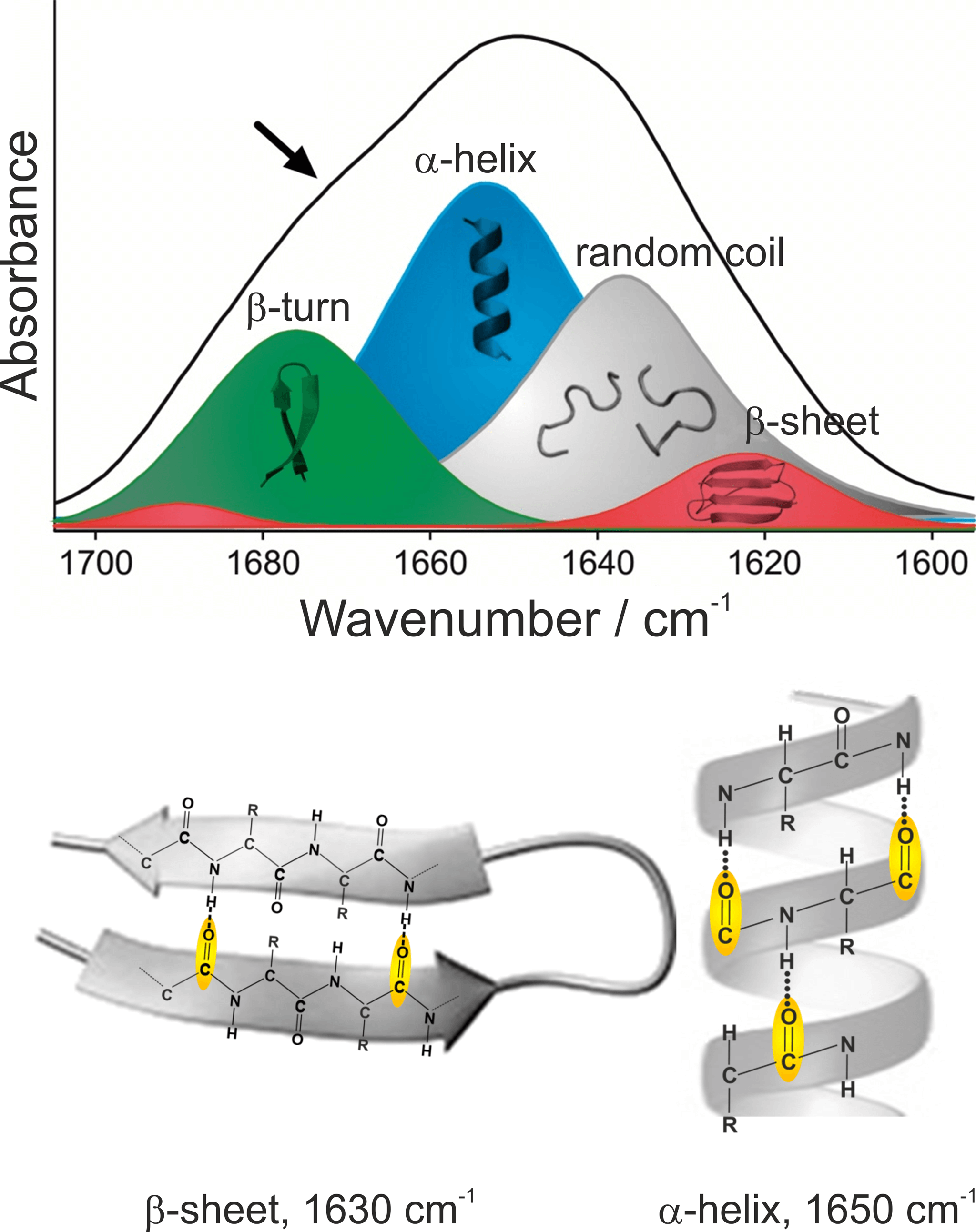
Figure 1: Assignment of secondary structural elements to their spectroscopic properties
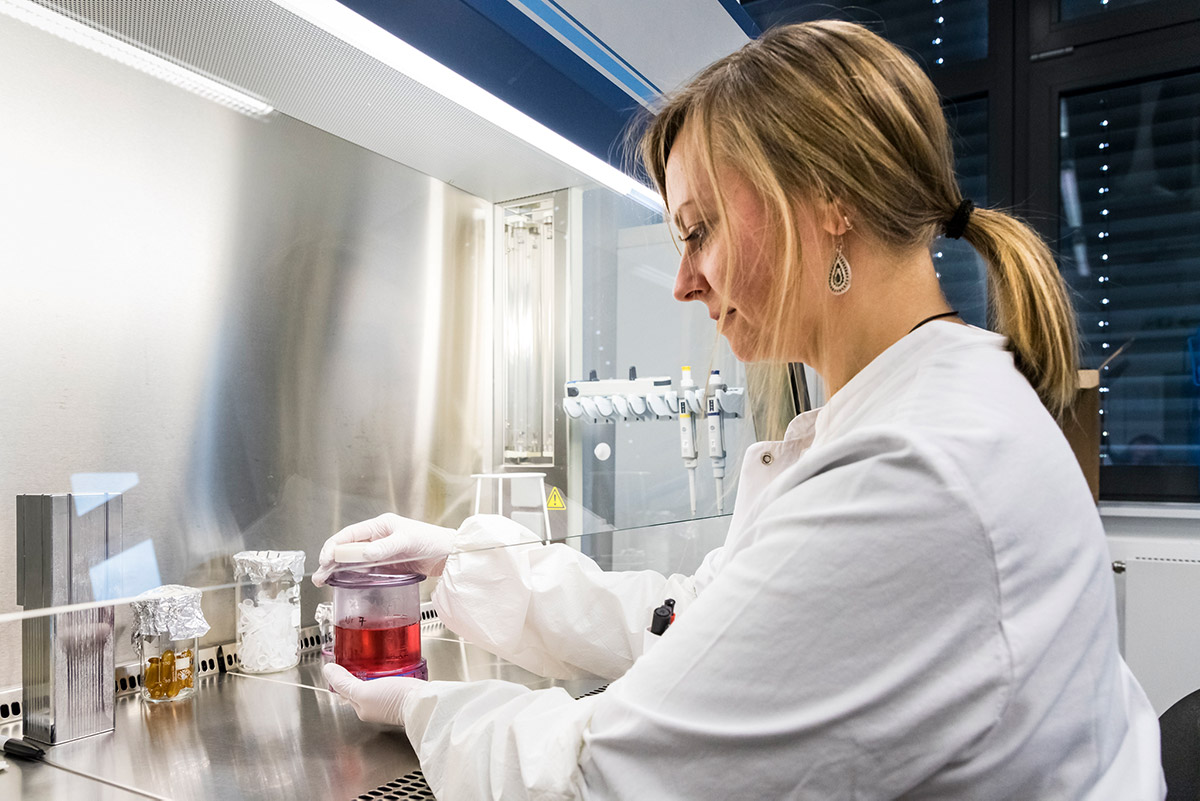
Figure 2: Medium change during the production of monoclonal antibodies.
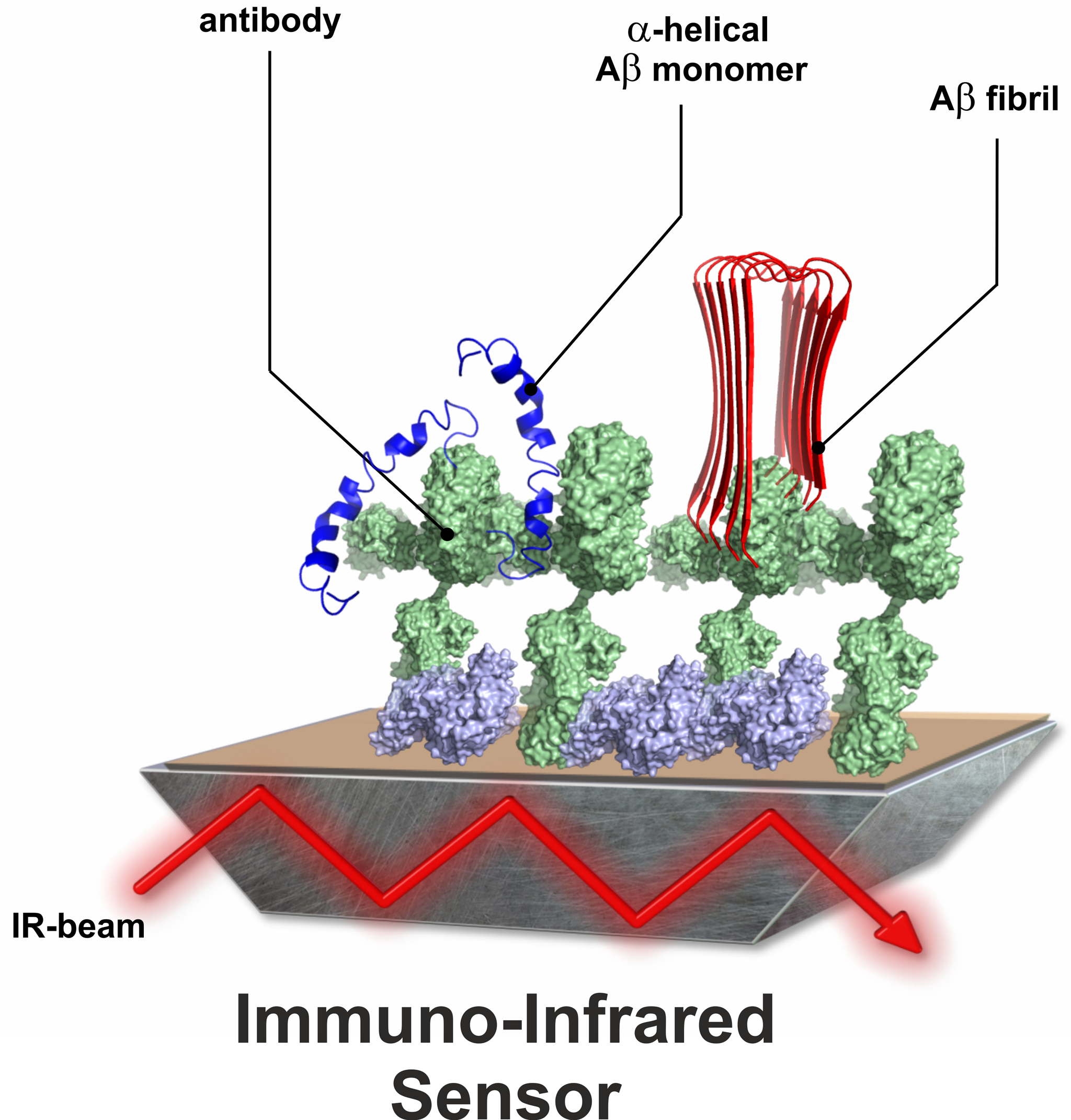
Figure 3: Schematic structure of the immuno-infrared sensor.
Immuno-infrared sensor for early diagnosis of Alzheimer’s disease
To enable the simultaneous detection and spectroscopic analysis of the secondary structure of Aβ-peptides, we have adapted our conventional spectroscopic methods and developed an immuno-infrared sensor. At the heart of this sensor is an antibody-coated surface of the internal reflection element (IRE) of an attenuated total reflection (ATR) assembly. In the ATR setup, an infrared beam is passed through the IRE (Nabers et al Journal of Biophotonics 2016). The IR beam evanescing on the surface of the IRE interacts with proteins immobilised on this surface via specific antibodies. It is crucial that the antibody used binds all conformations of the protein in parallel and thus the secondary structure distribution can be determined.
By differentiating the spectra before and after binding of the target protein, the secondary structure-sensitive amide-I band of the protein backbone of the target protein is measured. The detected amide I band represents an integrated signal over all bound protein conformations. The higher the proportion of pathogenic forms in the overall distribution, the further the maximum of the detected amide-I band shifts to lower wavenumbers. The β-sheet-rich toxic isoforms show a maximum in the region around 1638 cm-1, whereas monomeric non-toxic forms absorb at around 1652 cm-1. Our studies have shown that in healthy subjects the spectroscopic maximum of the amide I band is above an experimentally determined threshold range around 1643 cm-1, whereas in subjects with Alzheimer’s disease it is below this threshold. In previous studies, our immuno-infrared sensor has predicted the correct trend in patients who later developed Alzheimer’s disease up to 8 years before clinical manifestation of symptoms (Nabers et al EMBO Molecular Medicine 2018). The use of novel quantum cascade lasers (QCL) as an infrared light source now offers the possibility of optimising and miniaturising the measurement setup. This is the first step in the development of a device suitable for clinical use.
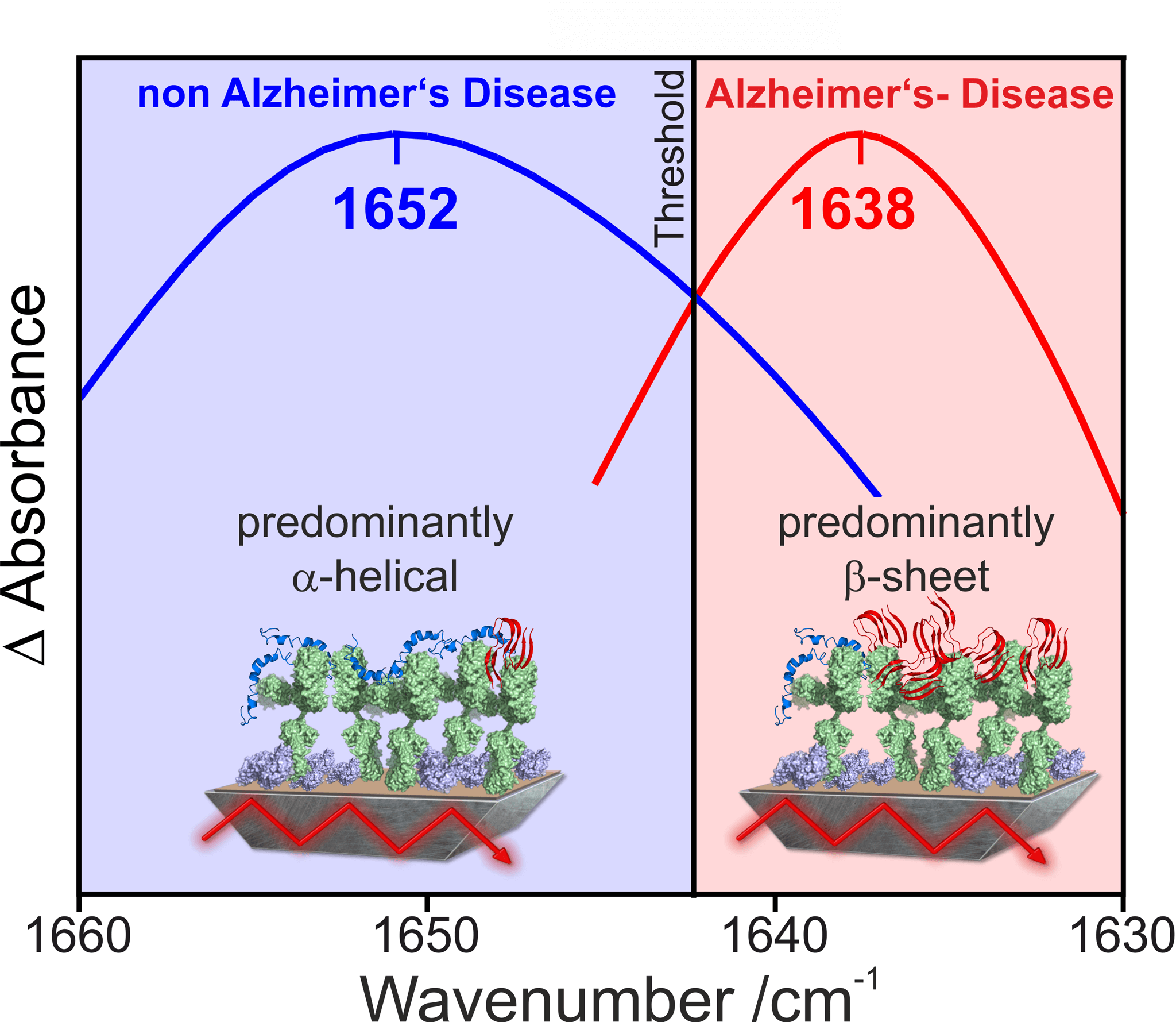
Figure 4: Spectral differentiation between patients with Alzheimer’s and healthy people
Press
Press releases
Press review
External Funding
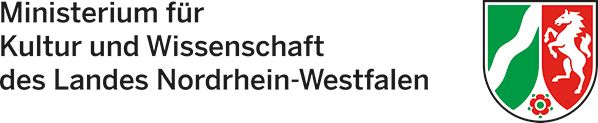
111.08.03.05-133974 Validierung und Standardisierung des Workflows zur Proteindiagnostik
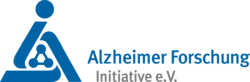
Correlation between Aβ42 decrease and misfolding in blood
131/1.08-031 PURE – Protein Research Unit Ruhr within Europe
233-1.08.03.03-031-68079 Validierung Marker-freier Imaging Verfahren und neu identifizierter Biomarker unter Nutzung des PURE-Konsortium